The O’Doherty Group Research Summary:
Summary of Results: The O’Doherty research group has been developing new methods for the de novo synthesis of natural and unnatural structures, with a particular focus on the synthesis of biologically active natural products and carbohydrate motifs. Our overarching synthetic goal is to use asymmetric transition metal catalysis to create absolute stereochemistry and a combination of catalysis and substrate control for diastereocontrol. This concept can be seen in several of our natural product syntheses (Schemes 1-7 and 11-14) as well as our expanding ability to synthesize carbohydrates (Schemes 8-24). For example our early efforts to control all the stereochemistry of monosaccharides led to using catalysis to control the stereochemistry of the glycosidic bond and ultimately complex oligosaccharides. A long-term theme to this research is the development of highly stereoselective transformation that can be used for not only synthesis but also to enable medicinal chemistry studies. In fact, we have already had great success along these lines (vide infra).
I. Natural Product Synthesis:
a) The Pyranone Natural products: Our early efforts to use asymmetric synthesis of biologically relevant natural products began with our use of both the Noyori reduction and the Sharpless dihydroxylation reaction for the synthesis of optically pure furan alcohols (Scheme 1). These furan alcohols, via the Achmatowicz oxidation, could be converted into various pyranone natural products. Examples of these target molecules were the altholactone class of pyranone natural products 1.1, which naturally lead us to investigate a synthesis of various members of the phomopsolides 1.12.[i],[ii] Our want for a more atom economical approach to the Phomopsolides lead to a synthesis of Phomopsolide E dienyl acylfuran 1.13, which eventually led to the discovery of new dienoate oxidation and hydration chemistry for both natural product and carbohydrate synthesis.[iii]
Scheme 1: A furan dienoate approach to the antifungal pyranones Phomopsolide D and E
b) The Polyol/Pyranone Natural Products: Our experience with the Altholactones and Phomopsolides led to our interest in studying the Cryptocarya polyacetates, which are 1,3-polyol/pyranone natural products (Scheme 2). Because of the repeating 1,3-syn-polyol portion of this class of natural products, we developed an iterative hydration methodology for the de novo synthesis of 1,3-diols from achiral dienoates (2.6 from 2.7).[iv] Using a related iterative oxidation of trienoate (2.11 to 2.10) lead to a synthesis of the antitumor pyranone natural product Anamarine and its C-11 epimer.[v] The recognition of the selective trienoate chemistry led us to try using the asymmetric hydration chemistry of trienoates and more complex natural products, like the colletodiols.
Scheme 2: Synthesis of cyrptocary diacetate and related polyol /pyranone natural products
c) The Colletodiol Macrolactones: We have also expanded our dienoate methodology to trienoates (3.8, Scheme 3). The power of this procedure is demonstrated in our 6-step route to bis-enoate 3.6, which became a key fragment of our synthesis of the bismacrolactone antibiotics colletodiols, colletoketol and colletol. These efforts recently culminated in an 11-step enantioselective synthesis (longest linear route) of colletodiol 3.3, 12-step synthesis of colletoketol 3.2 and 13-step synthesis of colletol 3.1. A key issue for the use of this methodology for convergent synthesis is the enantiopurity of the products. Fortunately, we have found that most of the diols are formed in high enantioexcesses (> 95 %ee).[vi]
Scheme 3: Synthesis of the colletodiol type bismacrolactone natural products from di- and tri-enoates
d) The Cladospolides: Our synthesis of the cladospolides had the same need for high enantiocontrol in the diastereocontrol introduction of distant chiral centers. This led us to explore the use of the Noyori ynoate reduction with the Sharpless asymmetric dihydroxylation reaction for the synthesis of (2,X,X+1)-triols (Scheme 4). This was accomplished by recognizing that a combination of the alkyne zipper reaction with the Trost dienoate dienoate synthesis could relay the required functionality across the eight carbon atoms (4.6 to 4.4).[vii]
Scheme 4: De novo approach to Cladospolide B-D
e) Apicularen A: Our success with the use of the iterative hydration of dienoate chemistry, led us to want to explore its use for the synthesis of more complex 1,3-polyol natural products. These efforts led to a formal de novo synthesis of Apicularen A, which used the iterative hydration chemistry in combination with a transannular hydration reaction (Scheme 5).
Scheme 5: De novo approach to (–)-Apicularen A
f) Milbemycin b3: Our interest further exploring the scope of this hydration reaction chemistry also lead to a highly efficient synthesis of Milbemycin b3. Again, this route used our bis-hydration of dienoates as well as the hydration of a substituted dienone to set all the chiral centers of the spiroketal portion of the natural product (Scheme 6).
Scheme 6: De novo Synthesis of Milbemycin b3
g) RK-397: A combination of our asymmetric dienoate hydration reactions has led to a formal synthesis of the anti-tumor antibiotic RK-397. This convergent synthesis also uses the Leighton allylation reactions, which we are currently looking to replace with Krische allylation reactions.
Scheme 7: De novo approach to RK-397
h) Summary of Results: So far we have shown that a de novo asymmetric approach to natural products allows for easy entry into various classes of biologically important structures. In addition to providing access to a range of structures, these approaches provided the first access to several of these natural products (e.g., Phomopsolide C-E, Cryptocarya triacetate, Cryptocarya tetraacetate, Cryptocaryolone, Cryprtocaryolone diacetate, Cladospolide D-E).
II. Carbohydrate Synthesis:
a) Overview: As with our natural product total synthesis, our initial goal was to use asymmetric transition metal catalysis to create all the stereochemistry of monosaccharides, in addition to using catalysis to control the stereochemistry of the glycosidic bond and thus complex oligosaccharides. A long-term theme to this research is the development of highly stereoselective glycosylation and post glycosylation transformations that can be used in diverse complex oligosaccharide settings (i.e., to be as reliable and predictable as the carbohydrate protecting group chemistry it replaces). In fact, we have already had great success in the preparation of common and uncommon monosaccharides and more recently have had success in extending this methodology toward the preparation of biologically important oligosaccharides (vide infra).[viii],[ix],[x] In this radical approach, the absolute D-/L-stereochemistry of a sugar is established by a highly stereoselective Noyori reduction of an acylfuran and the relative stereochemistry a-/b-stereochemistry is installed by a highly stereoselective Pd-catalyzed glycosylation reaction. This de novo approach initially transfers a pyranone ring (i.e., aculose) to the glycosyl acceptor where the enone functionality de facto serves as protecting groups for various sugar diastereomers (vide infra).
Scheme 8: De novo approach to the hexoses
b) De Novo Synthesis of Monosaccharides: Our initial approach to the various hexoses relies on an oxidative rearrangement of furfuryl alcohols to pyranones (Achmatowicz reaction[xi]). These furfuryl alcohols were produced via asymmetric catalysis (Sharpless oxidations/Noyori reductions). We have succeeded in developing a short route that is flexible enough for the synthesis of four of the eight possible diastereomeric hexoses and various deoxy- and aminosugars[xii] as either enantiomer (Scheme 8).[xiii] In addition, we have also developed an enantioselective route to galacto– and gluco-sugars[xiv] via a sequential bis-asymmetric dihydroxylation of dienoate 8.5.[xv],[xvi] By using substituted furans and dienoates this approach can be used for the synthesis of various stereoisomers of the papulacandins 8.2 (gluco-, manno– and allo-; Scheme 8).13,[xvii]
Scheme 9: Palladium catalyzed a/b-selective glycosylation
c) Glycosylation/Cyclitolization of alcohols: More recently we have been able to expand the de novo synthesis of monosaccharides to di-, tri- and oligosaccharides (vide infra). Key to the success of this approach is the development of a mild palladium catalyzed glycosylation (Scheme 9) in combination with the discovery of a highly enantioselective approach to pyranones from acylfurans via Noyori chemistry. As outlined in Scheme 9, the Pd-p-allyl catalyzed glycosylation reaction is both general and highly stereospecific using the C-2/C-3 p-bond as the anomeric directing group.[xviii],[xix] The reaction occurs rapidly and in high yields for both the a-9.3 and b-9.3. This reaction has great potential for preparing various D- and L-sugars because the starting 6-t-butoxycarboxy-2H-pyran-3(6H)-ones 9.3 can easily be prepared from optically pure furfuryl alcohols 9.2 (either (R) or (S) form)[xx] by a two-step procedure (Scheme 9). Depending on the reaction temperature of the second step, the acylation reaction can selectively give the a-Boc pyranones 9.3 at –78 °C; whereas at room temperature, a 1:1 ratio of the a- and b-Boc protected enones were produced. Once the pyranone (aculose) ring is installed the enone functionality can be transformed into various sugar diastereomers (Scheme 10). This can be seen in our syntheses of homo-adenosine and homo-deoxy adenosine (10.9a/b, which have been sent to NCI for testing).[xxi]
Scheme 10: Palladium catalyzed glycosylation reaction of oxygen and nitrogen nucleophiles
In fact, this approach has been expanded to include a cyclitol installation reaction (cyclitolization, 10.13 to 10.14). A key advantage of this de novo methodology is that the Pd-catalyzed glycosylation does not require the ring oxygen. So, it can be used for the installation of in-ring C-glycosides, cyclitolization. For example, the C-mannose (rhamnose) analogue 10.16 was prepared from the a-D-enone 10.14a-D via an analogous palladium catalyzed ionization/benzyl alcohol coupling (~1.3 equiv). The required enone 10.14a-D was easily prepared from quinic acid 10.10 via a route that is also amenable for the preparation of the other possible stereoisomers (e.g., b-D, a-L, b-L). In fact, we have prepared both enantiomers of the cyclitol analogues of SL0101 and Novobiocin (Schemes 12, 13, and 15).
d) De Novo Synthesis and SAR-study of Carbohydrate Natural Products: We have used the same de novo strategy to prepare nine carbohydrate-based natural products. This list includes the pheromone daumone, the RSK inhibitor SL0101, anticancer/antiviral agent swainsonine, as well as, the trisaccharide digoxose and its related cardio-toxin digitoxin (Schemes 11-20). These routes have provided significant amounts of material for biological testing and SAR studies, allowing access to analogues that are not readily available by traditional carbohydrate methods (e.g., regioisomers, diastereomers and enantiomers).
i) Synthesis of Daumone: This highly enantio- and diastereocontrolled method has been applied to a de novo synthesis of daumone (Scheme 11).[xxii] This approach provided significant quantities of daumone 11.1 as well as several analogues for mechanism of action studies. Early results from these studies showed that daumone accumulates within the cuticle of the pharynx and in the first intestinal cell.[xxiii]
Scheme 11: Synthesis of Daumone
ii) Synthesis of SL0101: We have also used both our Pd-glycosylation and cyclitolization for the synthesis of several potent RSK2 inhibitors based on the SL0101 natural product (12.4a).[xxiv] These routes provided material for mechanism of action studies. Testing the enantiomer D-12.4a showed the importance of the sugar to the molecules pharmacophore. Similarly, the improved activity of the cyclitol analogue 12.4a (X = CH2) suggests that hydrolysis of the glycosidic bond might be an issue for the natural product.
Scheme 12: Synthesis of both enantiomers of the potential antitumor glycoside SL0101 and cyclitol analogue
iii) Synthesis of Jadomycin A and B: We have also used our cyclitolization for the synthesis of a cyclitol analogue of the anti-tumor natural product Jadomycin B (13.10, X= CH2). These efforts began with the first successful synthesis of Jadomycin A 13.6. Interestingly, due to the instability of the natural O-glycoside 13.10 (X = O) to the acid oxazolone synthesis this structure still has not succumbed to total synthesis.[xxv]
Scheme 13: Synthesis of the cyclitol analogue of the potential antitumor natural product Jadomycin
iv) Synthesis of Swainsonine: We have also used this de novo asymmetric approach for the syntheses of swainsonine (14.9), its enantiomer (ent)-14.1 and several diastereomers (14.8-14.10).[xxvi],[xxvii] This highly enantio- and diastereocontrolled route illustrates the utility of the Noyori reduction, Achmatowicz reaction and palladium catalyzed glycosylation for natural product synthesis. This approach provided both enantiomers of swainsonine in 17% overall yield from furan 14.7. In addition to demonstrating the stereochemical flexibility, we desired both enantiomers, because the D-isomer is a known mannosidase inhibitor, whereas the L-isomer is a rhamnosidase inhibitor.
Scheme 14: Synthesis of D- or L-Swainsonine
e) Synthesis of Carbohydrate based Libraries of Natural Products
i) De Novo Glycosylation of Methymycin: As part of our efforts to prepare new antibiotics as well as to find reversible substrates for glycosyltransferases, we have performed the glycosylation/post-glycosylation on the macrolactone aglycon, 8,9-dihydro-10-deoxy-methymycin 16.1 (Scheme 16). The Hung-wen Liu lab at U-T Austin has shown 16.1 to be a substrate for the reversible glycosylation with methymycin transferase, therefore, establishing the precedent for our proposed macrolide library synthesis.
Scheme 16: Glycosylation of the macrolactone Methymycin
ii) Synthesis of Digitoxin: Our most successful use of this approach has been to alter the sugar portion of digitoxin. In fact we have had some success at using our de novo methodology for the synthesis and biological evaluation of glycosylated digitoxin based anticancer compounds (Scheme 17).[xxviii] This program was aimed at performing unique SAR studies on the carbohydrate portion of the molecule (Figures 1 and 2). Towards these ends, we have prepared and screened the mono-, di- and tri-saccharide digitoxin O– and neo-glycosides (X = N(OMe)) and showed that the O-linked mono- and tri-saccharides have nearly identical and potent cytotoxicity whereas the di-saccharide has reduced activity in a series of cell lines. These O-glycosides were also significantly more active than the neo-glycosides. These trends have emboldened us to pursue the synthesis and testing of a library of monosaccharides with both D-/L- and a-/b-stereochemistry (Scheme 17, Dig 4-10).
Scheme 17: Synthetic studies toward digitoxin
Figure 1: Apoptosis assay of Digitoxin vs Dig 6 (GJ22) Figure 2: Percent apoptosis
Mode of action studies in my colleague Prof. Rojanasakul’s lab in the school of pharmacy at WVU, indicates that these new mono-saccharide digitoxin analogues also kill cells by an apoptosis mechanism (Figure 1 and 2). Quantification of the degree of apoptosis indicates that the Dig 6 (GJ22) is about ten times as potent as digitoxin, and is capable of inducing apoptosis even in the low nanomolar range. We have determined that this apoptosis is caspase dependent, as evidenced by the lack of apoptosis in the wells pre-treated with ZVAD (a pan-caspase inhibitor). Both digitoxin (DTX) and the monosaccharide analogue (GJ22, Dig 6) inhibit the formation of tubules in a Human umbilical cord vascular endothelial cells model for angiogenesis with the monosaccharide showing a greater degree of capillary regression after 24 h treatment at 100nM.
iii) Anthrax tetrasaccharide for vaccines and detection: We have successfully applied our De Novo chemistry towards an even more complex oligosaccharide (Schemes 18-23).[xxix] For instance, the Anthrax tetrasaccharide 18.12, made up of three L-rhamnose sugars and a rare sugar called D-anthrose was synthesized.[xxx],[xxxi] The uniqueness of the anthrose sugar and the resistance of carbohydrate structures to evolutionary change, has attracted a lot of interest for the preparation of vaccines and detection devices based on the anthrax tetrasaccharide 18.12.[xxxii] Currently, we are testing the deprotected material from di- and trisaccharides 18.5 and 18.9 to elucidate the precise structural details of this detection.
Scheme 18: Structure relevant need for an anthrax detector
iv) Cleistetroside: Using a related approach as above (Scheme 18), two Cleistetrosides and one Cleistetrioside have been prepared (Scheme 19). This synthesis confirmed that this class of natural oligo-rhamnosides are L-rhamno-based. Because this is a highly efficient linear synthesis, we believe we will be able to make all the members of this class of compounds for testing. In fact, we already have 50 mg each of the first three targets (19.7, 19.8 and 19.9). Key to the success of this material is the mild nature of our glycosylation/postglycosylation reactions that allows for the direct incorporation of acid and base sensitive acetate and chloro-acetate groups, which allow for the synthesis of all the members of this family (Schemes 19).[xxxiii]
Scheme 19: Synthesis of the 1,4/1,4-linked tetra-rhamno-sugar Cleistetrosides
v) Landomycin E trisaccharide: We have also investigated the use of our de novo methodology for the synthesis of other biologically related trisaccharides (Schemes 20 and 21). These efforts have culminated in the synthesis of the trisaccharide portion of two Aquayamycin antitumor antibiotics, landomycin E and PI-080.[xxxiv] Key to the success of this approach was the discovery of a highly regioselective Mitsunobu-like inversion of the axial alcohol of a cis-1,2-diol (20.3 to 20.4 and 20.6 to 20.7). This transformation in combination with a highly diastereoselective dihydroxylation reaction becomes a nice solution to the problem of 1,2-trans-diequatorial addition to a cyclohexene. Current efforts are now focused on the selective dimerization of the trisaccharide 20.9 to the full landomycin E hexasaccharide and its binding to DNA.
Scheme 20: Synthesis of the trisaccharide portion of Landomycin A
vi) PI-080 trisaccharide: The same selective Mitsunobu inversion/diol protection (21.3 to 21.4) was used in the synthesis of the trisaccharide portion of PI-080. With significant quantities of trisaccharide 21.8 in hand, we decided to screen it against a panel of cancer cell lines from nine different tissue types. While we were motivated to test 21.8 primarily as a control for future studies, we were delighted to find it had wide ranging cytotoxicity, displaying significant growth inhibition (GI50 from 0.1 to 11 mM) and cytotoxicity (LC50 from 5.1 to 100 mM) against these various cell lines. In fact, at 50 mM, the trisaccharide 21.8 showed greater cytotoxicity than Cisplatin at 300 mM (Figure 3) and at 50mM this occurred via apoptosis (Figure 4). Interestingly, this level of cytotoxicity is in the range that is found for other glycosylated angucycline antibiotics.[xxxv]
Scheme 21: Synthesis of the trisaccharide portion PI-080
Figure 3. Cytotoxicity of 21.8/Cisplatin towards H460 Figure 4. Mechanism of cell death apoptosis/necrosis
f) Pd-catalyzed oligosaccharide synthesis: In terms of total steps, the previous tri- and tetra-saccharides that we so far have prepared are invariably assembled in as many or fewer steps than more traditional carbohydrate routes, primarily due to their use of extensive protection/deprotection strategies; however, a traditional carbohydrate approach would not allow for the incorporation of unnatural L-sugars.[xxxvi] The greatest potential for this p-allyl-Pd catalyzed glycosylation will be the use of bi-directional synthesis for the generation of biologically important natural and unnatural oligosaccharides. When this method is applied radically shorter routes are revealed and once again illustrating the role of the enone as a versatile triol protecting group.
i) 1,6-oligosaccharides: As outlined in Scheme 22, the 1,6-manno-trisaccharide 22.1bwas prepared from acylfuran 22.6 with a C–6 OTBS group. The synthesis was accomplished by a simple TBS-deprotection/glycosylation strategy to first prepare disaccharide 22.4 and then trisaccharide 22.4 by repeating the two steps. The manno-stereochemistry of 22.1b was installed by a highly stereoselective one-pot NaBH4reduction and one-pot tris-dihydroxylation. Thus in only two steps, nine stereocenters were set.
Scheme 22: Synthesis of 1,6-tri-manno-sugars
ii) 1,4-oligosaccharides: A similar strategy was used for the preparation of both the 1,4-manno-trisaccharide 23.5a and the 2,4-deoxy trisaccharide 23.5b, as well as one of the glycosylated peptides required for Mannopeptimycin (Scheme 23).[xxxvii] Our route to the trisaccharides started with enone 23.1, which was prepared from the furfuryl alcohol in 2 steps. Finally, a tris-dihydroxylation reaction installed the remaining C-2/C-3 hydroxyl groups with manno-stereochemistry. Key to the success of this sequence was the highly stereoselective reduction and dihydroxylation reaction, which installed six stereocenters in one transformation.[xxxviii]
Scheme 23: Synthesis of 1,4-tri-manno-sugars
g) Summary: We have shown that a de novo asymmetric approach to carbohydrates (mono- to oligosaccharides) as well as to carbohydrate based natural products allows for an easy entry to sufficient quantities of complex molecules as well as various analogues for testing. We believe that these de novo approaches will enable medicinal chemists to more easily perform SAR studies on complex carbohydrate structures. These results also demonstrate our commitment to making significant quantities of material available to our collaborators, as well as, our willingness to return to the synthesis to make revised material. These new approaches to carbohydrates provide a practical access to unique complex oligosaccharides that are not readily accessible by traditional carbohydrate routes.
[i] For a review of our approach see: “Achmatowicz Approach To 5,6-Dihydro-2h-Pyran-2-One Containing Natural Products” in Strategy and Tactics in Natural Product Synthesis, Michael Harmata Ed., by Joel M. Harris, Miaosheng Li, Jana G. Scott and George A. O’Doherty” ELSEVIER, London, 2004.[ii] J. M. Harris and G. A. O’Doherty, Tetrahedron Lett. 2002, 43, 8195-8199. [iii] M. Li, and G. A. O’Doherty, Tetrahedron Lett. 2004, 45, 6407-6411. [iv] T. J. Hunter and G. A. O’Doherty Org. Lett. 2001, 3(7); 1049-1052. [v] D. Gao and G. A. O’Doherty, unpublished results. [vi] T. J. Hunter and G. A. O’Doherty, Org. Lett, 2002 4, 4447-4450. [vii] Y. Xing and G. A. O’Doherty, Org. Lett. 2009, 11, 1107–1110. [viii] Comely, A. C.; Eelkema, R.; Minnaard, A. J.; Feringa, B. L., J. Am. Chem. Soc. 2003, 125, 8714-8715. [ix] For other approaches to palladium p-allyl glycal intermediates see: a) Dunkerton, L. V.; Serino, A. J. Carbohydrate Res. 1987, 171, 89-107. b) RajanBabu, T. V., J. Org. Chem. 1985, 50, 3642. [x] Mc Donald has a related non-p-allyl approach to oligosaccharides, see: McDonald, F. E.; Zhu, H. Y. H., J. Am. Chem. Soc. 1998, 120, 4246-4247. [xi] For a good review: (a) Zamoiski, A., Banaszek, A.; Grynkiewicz, G. Advances in Carbohydrate Chemistry and Biochemistry 1982, 40, 1. (b) Achmatowicz, O. ; Bielski, R. Carbohydr. Res. 1977, 55, 165-176. (c) Grapsas, I., K.; Couladouros, E. A.; Georgiadis, M. P., Pol. J. Chem. 1990, 64, 823-826. [xii] M. H. Haukaas and G. A. O’Doherty, Org. Lett. 2001, 3, 3899-3992. [xiii] (a) Md. M. Ahmed and G. A. O’Doherty, Carbohydr. Res. 2006, 341, 1505-1521. (b) Md. M. Ahmed and G. A. O’Doherty, J. Org. Chem. 2005, 67, 10576-10578. (c) Md. M. Ahmed and G. A. O’Doherty, Tetrahedron Lett. 2005, 46, 4151-4155. (d) R. S. Babu and G. A. O’Doherty, J. Carb. Chem. 2005, 24, 169-177. (e) Y. Zhang and G. A. O’Doherty, Tetrahedron 2005, 61, 6337–6351. (f) Md. M. Ahmed and G. A. O’Doherty, Tetrahedron Lett. 2005, 46, 3015-3019. (g) Md. M. Ahmed, B. P. Berry, T. J. Hunter, D. J. Tomcik and G. A. O’Doherty, Org. Lett. 2005, 7, 745-748. [xiv] Md. M. Ahmed, B. P. Berry, T. J. Hunter, D. J. Tomcik and G. A. O’Doherty, Org. Lett. 2005, 7, 745-748. [xv] Md. Moinuddin Ahmed, Matthew S. Mortensen and George A. O’Doherty J. Org. Chem. 2006, 71, 7741-7746. [xvi] (a) Md. M. Ahmed, H. Cui and G. A. O’Doherty, J. Org. Chem. 2006, 71, 6686-6689. (b) Md. M. Ahmed and G. A. O’Doherty, Carbohydr. Res. 2006, 341, 1505-1521. (c) Md. M. Ahmed and G. A. O’Doherty, J. Org. Chem. 2005, 67, 10576 – 10578. (d) Md. M. Ahmed and G. A. O’Doherty, Tetrahedron Lett. 2005, 46, 3015-3019. See, ref. 13. [xvii] (a) D. Balachari, and G. A. O’Doherty, Org. Lett. 2000, 2, 863-866. (b) D. Balachari and G. A. O’Doherty, Org. Lett. 2000, 2, 4033-4036. [xviii] Recently, both the poor reactivity in Pd catalyzed allylation reaction of alcohols as well as a nice solution to this problem was reported, see: Kim, H.; Lee, C. Org. Lett., 2002, 4, 4369. [xix] For a related Rh system, see: Evans, P. A.; Kennedy, L. J., Org. Lett. 2000, 2; 2213-2215. [xx] We have had great success at the preparation of optically pure furan alcohols from the Noyori reduction of the corresponding acylfuran, (vide infra). [xxi] S. R. Guppi, M. Zhou and G. A. O’Doherty, Org. Lett. 2006, 8, 293-296. [xxii] (a) H. Guo and G. A. O’Doherty, Org. Lett. 2005, 7, 3921-3924. [xxiii] T. J. Baiga, H. Guo, Y. Xing, G. A. O’Doherty, A. Parrish, A. Dillin, M. B. Austin, J. P. Noel, James J. La Clair, ACS Chem. Biol. 2008, ASAP. [xxiv] (a) M. Shan and G. A. O’Doherty, Org. Lett. 2006, 8, 5149-5152 [xxv] M. Shan, E. U. Sharif and G. A. O’Doherty, Angew. Chem. Int. Ed. ASAP. [xxvi] (a) H. Guo and G. A. O’Doherty, Org. Lett. 2006, 8, 1609 – 1612. [xxvii] H. Guo and G. A. O’Doherty, Tetrahedron, 2008, 64, 304-313. [xxviii] (a) M. Zhou and G. A. O’Doherty, Submitted. (b) M. Zhou and G. A. O’Doherty, Org. Lett. 2006, 8, 4339-4342. [xxix] H. Guo and G. A. O’Doherty, Angew. Chem. Int. Ed. 2007, 46, 5206-5208. [xxx] Daubenspeck, J. M; Zeng, H.; Chen, P.; Dong, S.; Steichen, C. T; Krishna, N. R.; Pritchard, D. G.; Turnbough, C. L. Jr. J. Biol. Chem. 2004, 279, 30945–30953. [xxxi] (a) Brachman PS, Kaufmann AF. Anthrax. In: Evans AS, Brachman PS, eds. Bacterial infections of humans. New York: Plenum Medical Book Company, 1998, 95-111. (b) Koch R. Med Classics 1937, 2, 787. (a) M. Mock, A. Fouet, Anthrax. Annu. Rev. Microbiol. 2001, 55, 647-671. (b) P. Sylvestre, E. Couture-Tosi, M. Mock, Mol. Microbiol. 2002, 45, 169–178. [xxxii] (a) Werz, D. B.;Seeberger, P. H., Angew. Chem. Int. Ed. 2005, 44, 6315-6318. (b) Adamo,R.;Saksena, R.; Kovac. P. Carbohydr. Res. 2005, 340, 2579-2582. (c) Wang, D.; Carroll, G. T.; Turro, N. J.; Koberstein, J. T.; Kovac, P; Saksena, R.; Adamo, R.; Herzenberg, L. A.; Herzenberg, L. A.; Steinman, L Proteomics 2007, 7, 180-184. [xxxiii] B. Wu, M. Li and and G. A. O’Doherty, Org. Lett. ASAP. [xxxiv] M. Zhou and G. A. O’Doherty, Org. Lett. 2008, 10. [xxxv] Abdelfattah, M. S.; Kharel, M. K.; Hitron, J. A.; Baig, I.; Rohr, J. J. Nat. Prod. 2008, ASAP. [xxxvi] (a) M. Zhou and G. A. O’Doherty, Org. Lett. 2006, 8, 4339-4342. (b) R. S. Babu, Sanjeeva R. Guppi and G. A. O’Doherty, Org. Lett. 2006, 8, 1605 – 1608. (c) R. S. Babu and G. A. O’Doherty, J. Carb. Chem. 2005, 24, 169-177. (d) R. S. Babu, M. Zhou and G. A. O’Doherty, J. Am. Chem. Soc. 2004, 126, 3428 – 3429. [xxxvii] R. S. Babu, Sanjeeva R. Guppi and G. A. O’Doherty, Org. Lett. 2006, 8, 1605-1608. [xxxviii] (a) M. Zhou and G. A. O’Doherty, Org. Lett. 2006, 8, 4339-4342. (b) R. S. Babu, Sanjeeva R. Guppi and G. A. O’Doherty, Org. Lett. 2006, 8, 1605 – 1608.
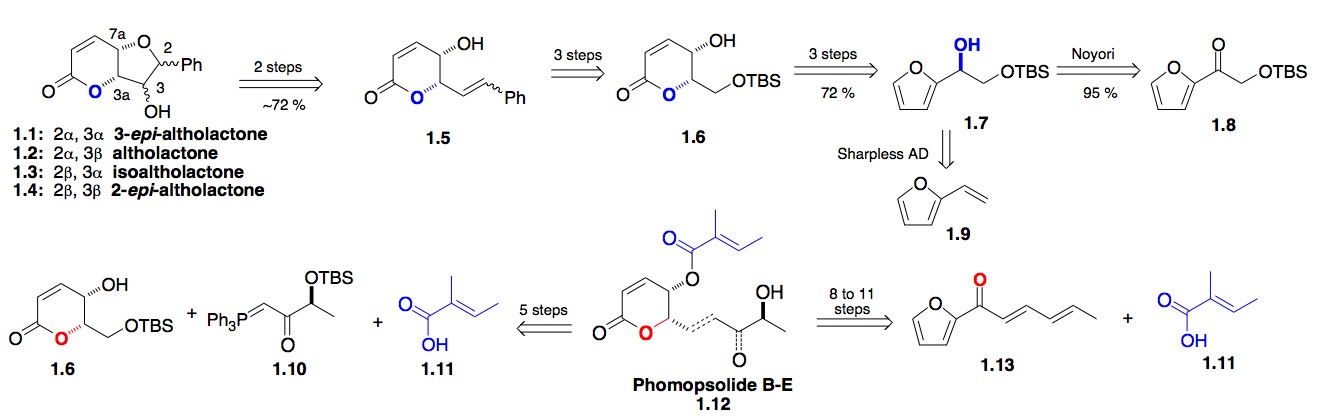
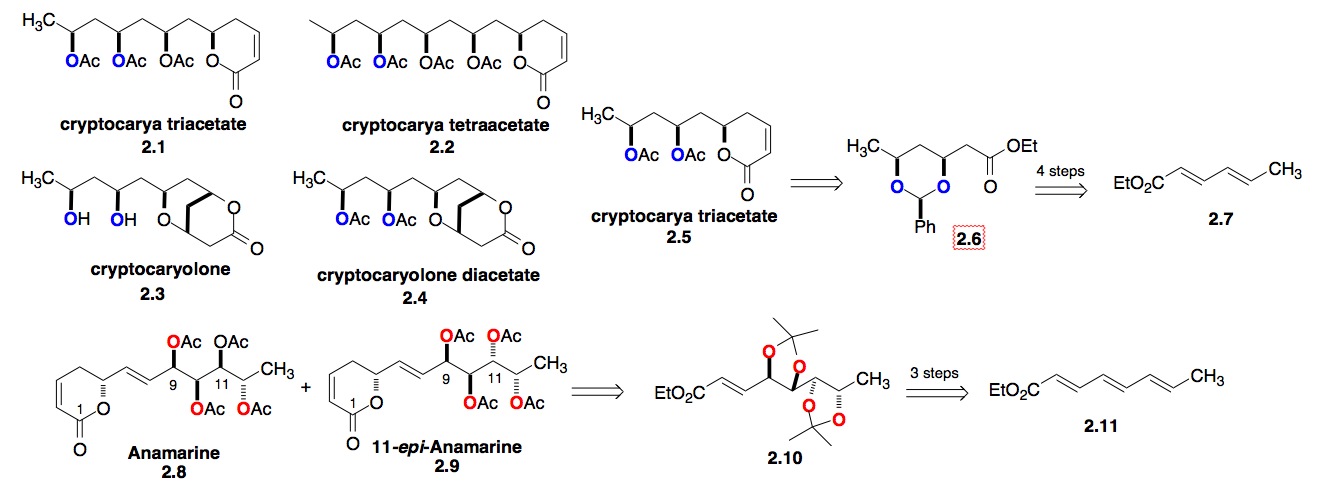
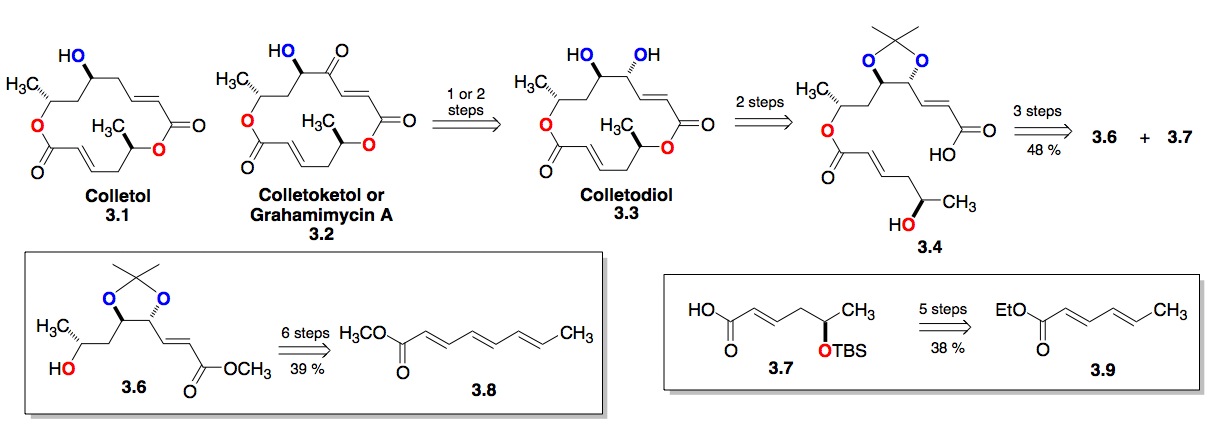
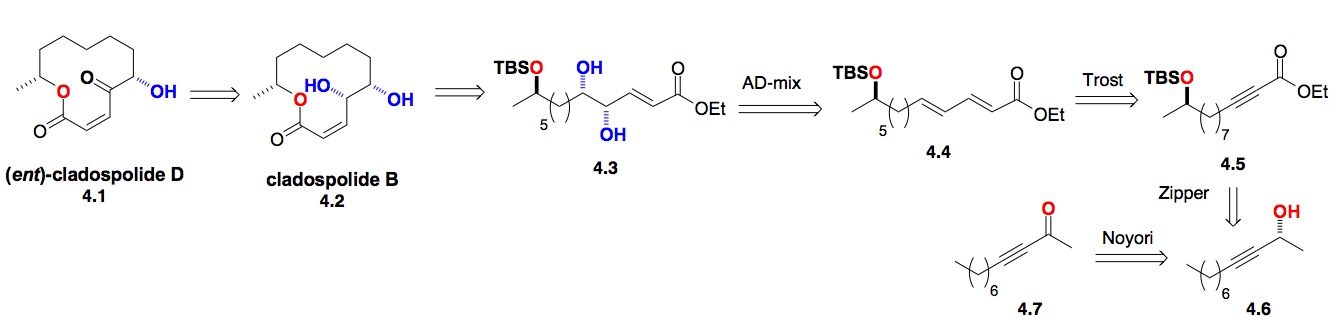
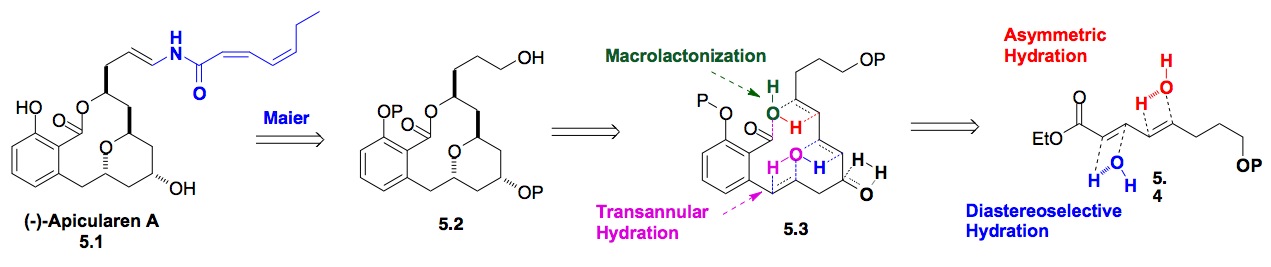
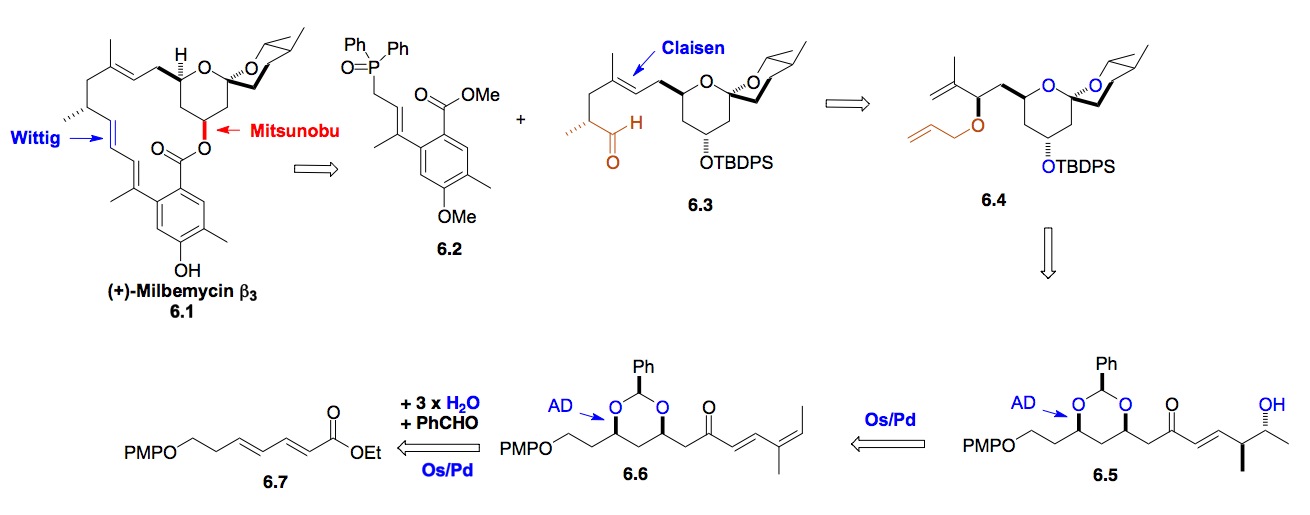
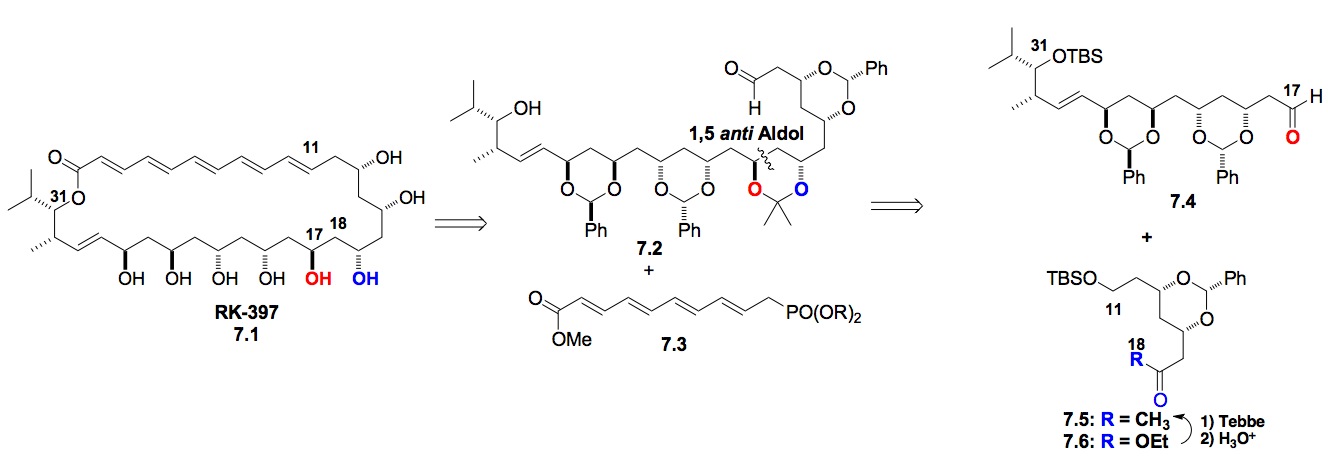
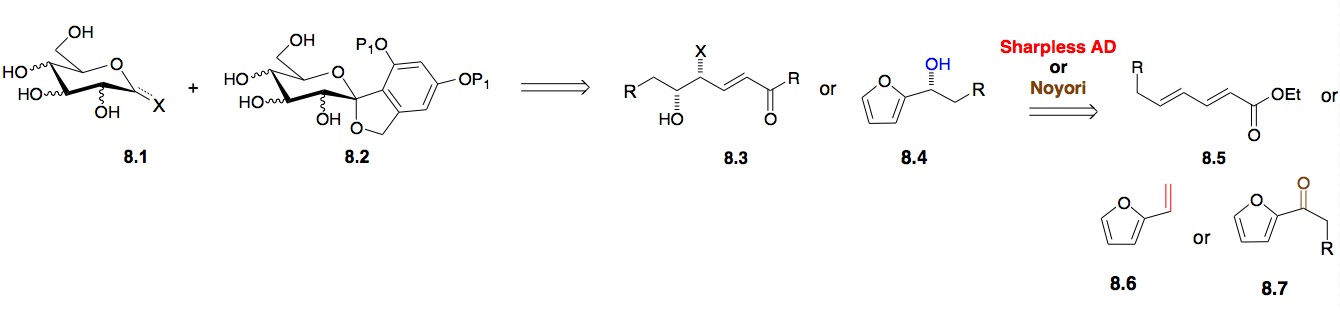
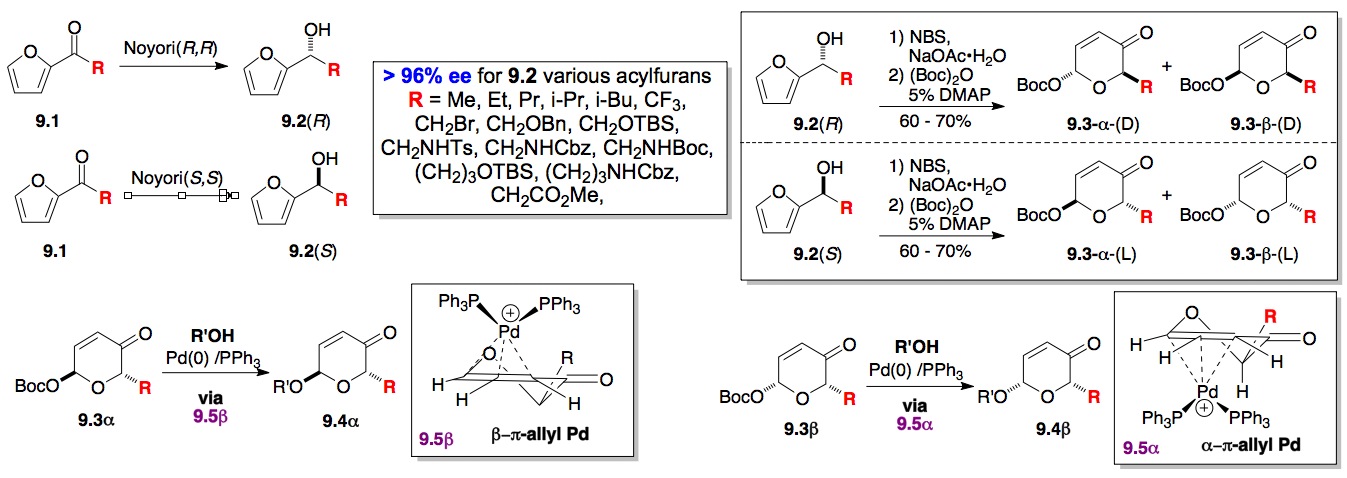
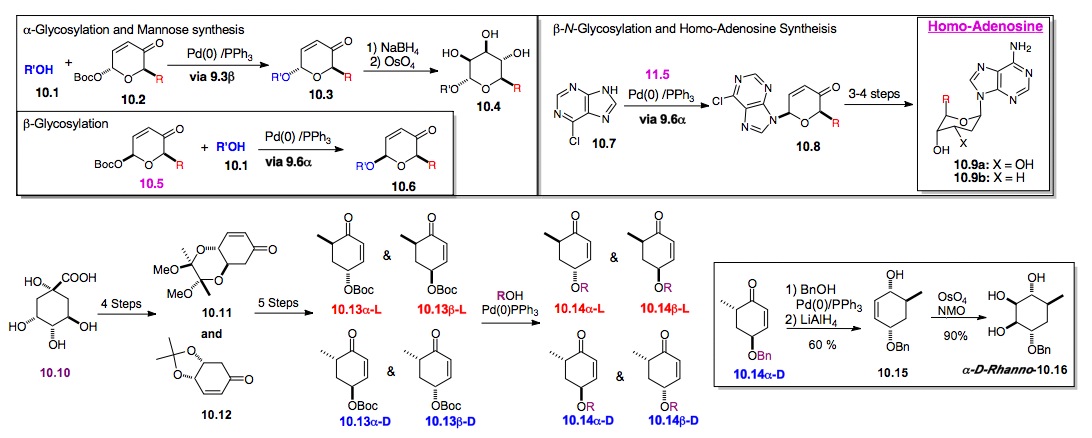


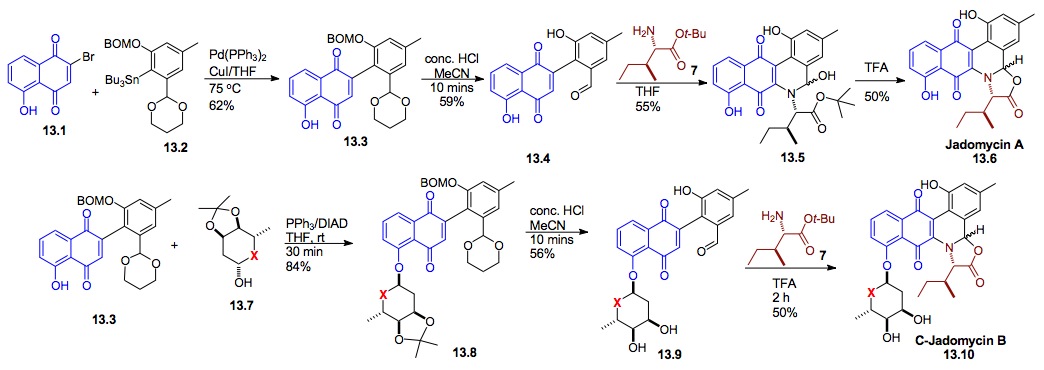
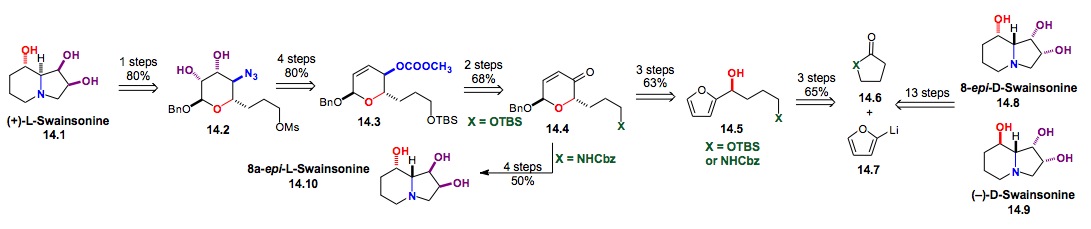
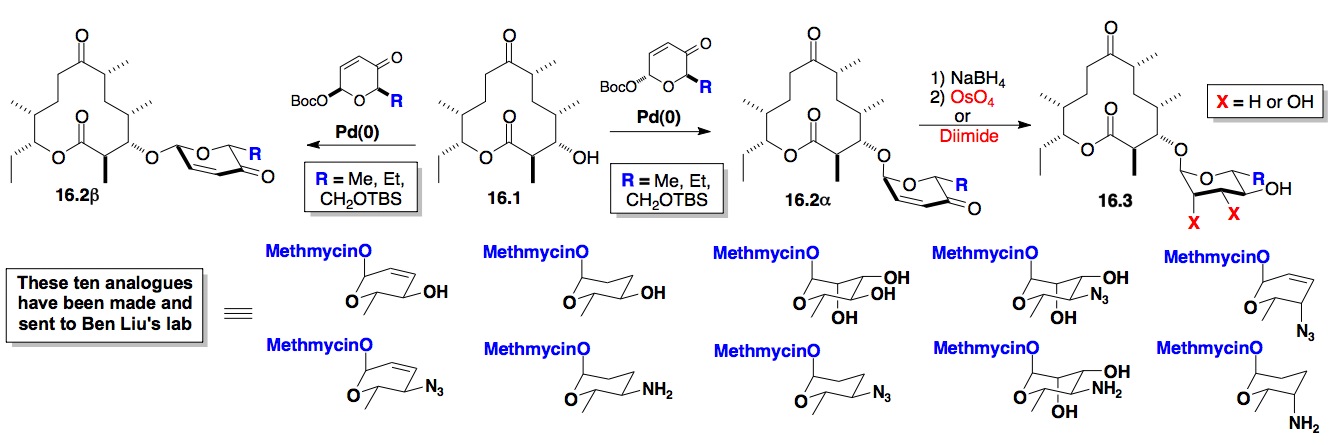
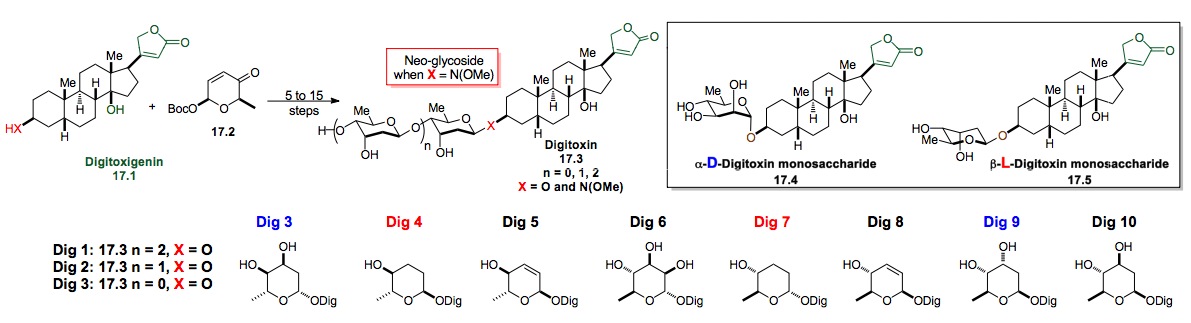
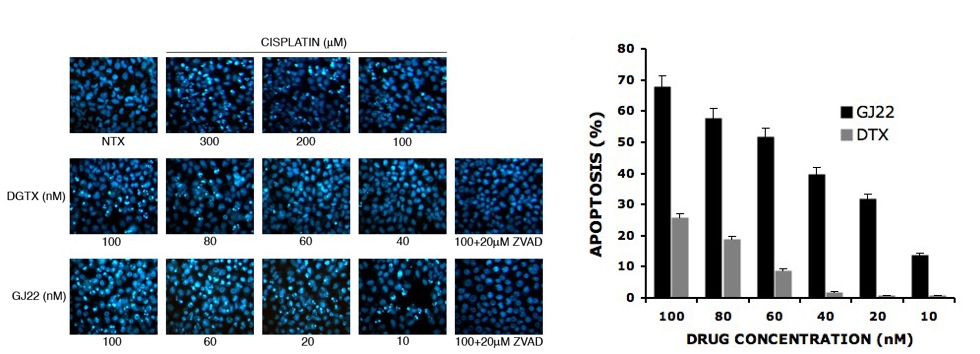
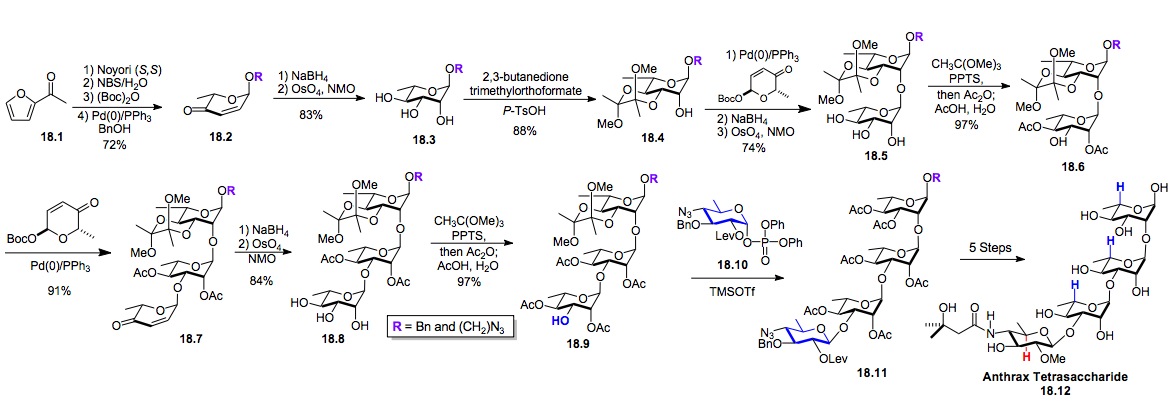

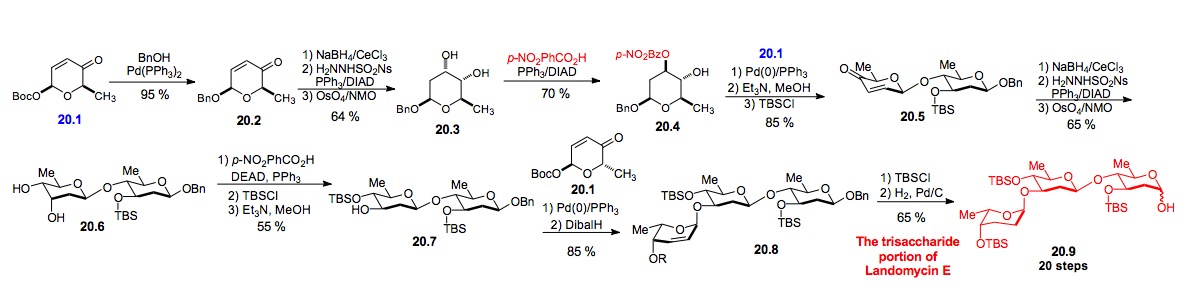
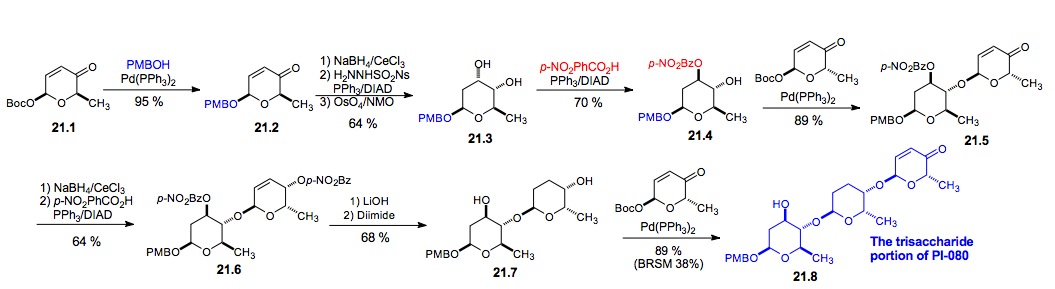
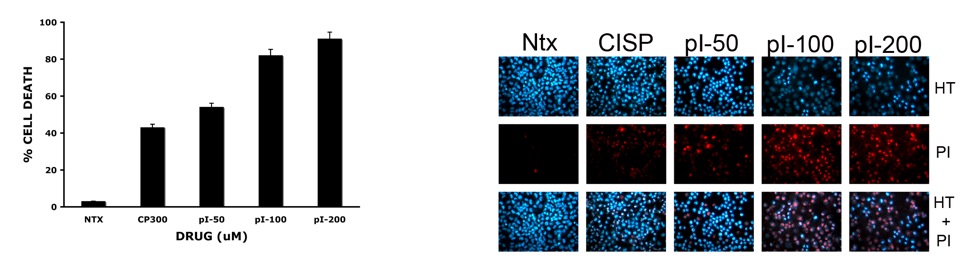
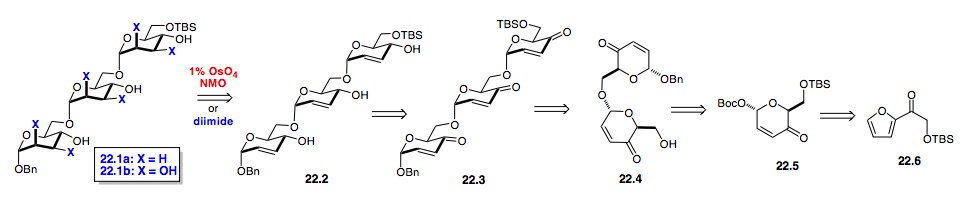
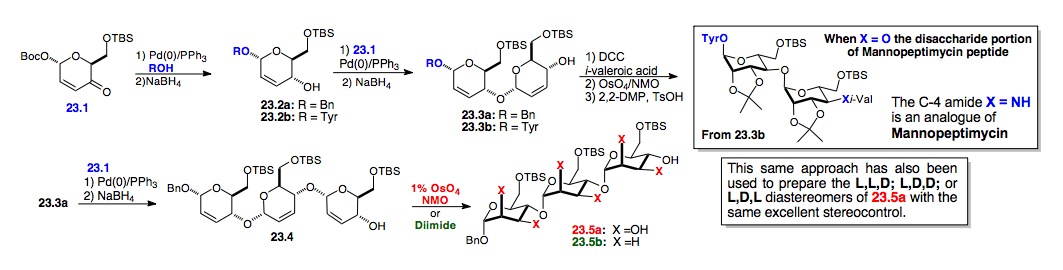
[i] For a review of our approach see: “Achmatowicz Approach To 5,6-Dihydro-2h-Pyran-2-One Containing Natural Products” in Strategy and Tactics in Natural Product Synthesis, Michael Harmata Ed., by Joel M. Harris, Miaosheng Li, Jana G. Scott and George A. O’Doherty” ELSEVIER, London, 2004.[ii] J. M. Harris and G. A. O’Doherty, Tetrahedron Lett. 2002, 43, 8195-8199. [iii] M. Li, and G. A. O’Doherty, Tetrahedron Lett. 2004, 45, 6407-6411. [iv] T. J. Hunter and G. A. O’Doherty Org. Lett. 2001, 3(7); 1049-1052. [v] D. Gao and G. A. O’Doherty, unpublished results. [vi] T. J. Hunter and G. A. O’Doherty, Org. Lett, 2002 4, 4447-4450. [vii] Y. Xing and G. A. O’Doherty, Org. Lett. 2009, 11, 1107–1110. [viii] Comely, A. C.; Eelkema, R.; Minnaard, A. J.; Feringa, B. L., J. Am. Chem. Soc. 2003, 125, 8714-8715. [ix] For other approaches to palladium p-allyl glycal intermediates see: a) Dunkerton, L. V.; Serino, A. J. Carbohydrate Res. 1987, 171, 89-107. b) RajanBabu, T. V., J. Org. Chem. 1985, 50, 3642. [x] Mc Donald has a related non-p-allyl approach to oligosaccharides, see: McDonald, F. E.; Zhu, H. Y. H., J. Am. Chem. Soc. 1998, 120, 4246-4247. [xi] For a good review: (a) Zamoiski, A., Banaszek, A.; Grynkiewicz, G. Advances in Carbohydrate Chemistry and Biochemistry 1982, 40, 1. (b) Achmatowicz, O. ; Bielski, R. Carbohydr. Res. 1977, 55, 165-176. (c) Grapsas, I., K.; Couladouros, E. A.; Georgiadis, M. P., Pol. J. Chem. 1990, 64, 823-826. [xii] M. H. Haukaas and G. A. O’Doherty, Org. Lett. 2001, 3, 3899-3992. [xiii] (a) Md. M. Ahmed and G. A. O’Doherty, Carbohydr. Res. 2006, 341, 1505-1521. (b) Md. M. Ahmed and G. A. O’Doherty, J. Org. Chem. 2005, 67, 10576-10578. (c) Md. M. Ahmed and G. A. O’Doherty, Tetrahedron Lett. 2005, 46, 4151-4155. (d) R. S. Babu and G. A. O’Doherty, J. Carb. Chem. 2005, 24, 169-177. (e) Y. Zhang and G. A. O’Doherty, Tetrahedron 2005, 61, 6337–6351. (f) Md. M. Ahmed and G. A. O’Doherty, Tetrahedron Lett. 2005, 46, 3015-3019. (g) Md. M. Ahmed, B. P. Berry, T. J. Hunter, D. J. Tomcik and G. A. O’Doherty, Org. Lett. 2005, 7, 745-748. [xiv] Md. M. Ahmed, B. P. Berry, T. J. Hunter, D. J. Tomcik and G. A. O’Doherty, Org. Lett. 2005, 7, 745-748. [xv] Md. Moinuddin Ahmed, Matthew S. Mortensen and George A. O’Doherty J. Org. Chem. 2006, 71, 7741-7746. [xvi] (a) Md. M. Ahmed, H. Cui and G. A. O’Doherty, J. Org. Chem. 2006, 71, 6686-6689. (b) Md. M. Ahmed and G. A. O’Doherty, Carbohydr. Res. 2006, 341, 1505-1521. (c) Md. M. Ahmed and G. A. O’Doherty, J. Org. Chem. 2005, 67, 10576 – 10578. (d) Md. M. Ahmed and G. A. O’Doherty, Tetrahedron Lett. 2005, 46, 3015-3019. See, ref. 13. [xvii] (a) D. Balachari, and G. A. O’Doherty, Org. Lett. 2000, 2, 863-866. (b) D. Balachari and G. A. O’Doherty, Org. Lett. 2000, 2, 4033-4036. [xviii] Recently, both the poor reactivity in Pd catalyzed allylation reaction of alcohols as well as a nice solution to this problem was reported, see: Kim, H.; Lee, C. Org. Lett., 2002, 4, 4369. [xix] For a related Rh system, see: Evans, P. A.; Kennedy, L. J., Org. Lett. 2000, 2; 2213-2215. [xx] We have had great success at the preparation of optically pure furan alcohols from the Noyori reduction of the corresponding acylfuran, (vide infra). [xxi] S. R. Guppi, M. Zhou and G. A. O’Doherty, Org. Lett. 2006, 8, 293-296. [xxii] (a) H. Guo and G. A. O’Doherty, Org. Lett. 2005, 7, 3921-3924. [xxiii] T. J. Baiga, H. Guo, Y. Xing, G. A. O’Doherty, A. Parrish, A. Dillin, M. B. Austin, J. P. Noel, James J. La Clair, ACS Chem. Biol. 2008, ASAP. [xxiv] (a) M. Shan and G. A. O’Doherty, Org. Lett. 2006, 8, 5149-5152 [xxv] M. Shan, E. U. Sharif and G. A. O’Doherty, Angew. Chem. Int. Ed. ASAP. [xxvi] (a) H. Guo and G. A. O’Doherty, Org. Lett. 2006, 8, 1609 – 1612. [xxvii] H. Guo and G. A. O’Doherty, Tetrahedron, 2008, 64, 304-313. [xxviii] (a) M. Zhou and G. A. O’Doherty, Submitted. (b) M. Zhou and G. A. O’Doherty, Org. Lett. 2006, 8, 4339-4342. [xxix] H. Guo and G. A. O’Doherty, Angew. Chem. Int. Ed. 2007, 46, 5206-5208. [xxx] Daubenspeck, J. M; Zeng, H.; Chen, P.; Dong, S.; Steichen, C. T; Krishna, N. R.; Pritchard, D. G.; Turnbough, C. L. Jr. J. Biol. Chem. 2004, 279, 30945–30953. [xxxi] (a) Brachman PS, Kaufmann AF. Anthrax. In: Evans AS, Brachman PS, eds. Bacterial infections of humans. New York: Plenum Medical Book Company, 1998, 95-111. (b) Koch R. Med Classics 1937, 2, 787. (a) M. Mock, A. Fouet, Anthrax. Annu. Rev. Microbiol. 2001, 55, 647-671. (b) P. Sylvestre, E. Couture-Tosi, M. Mock, Mol. Microbiol. 2002, 45, 169–178. [xxxii] (a) Werz, D. B.;Seeberger, P. H., Angew. Chem. Int. Ed. 2005, 44, 6315-6318. (b) Adamo,R.;Saksena, R.; Kovac. P. Carbohydr. Res. 2005, 340, 2579-2582. (c) Wang, D.; Carroll, G. T.; Turro, N. J.; Koberstein, J. T.; Kovac, P; Saksena, R.; Adamo, R.; Herzenberg, L. A.; Herzenberg, L. A.; Steinman, L Proteomics 2007, 7, 180-184. [xxxiii] B. Wu, M. Li and and G. A. O’Doherty, Org. Lett. ASAP. [xxxiv] M. Zhou and G. A. O’Doherty, Org. Lett. 2008, 10. [xxxv] Abdelfattah, M. S.; Kharel, M. K.; Hitron, J. A.; Baig, I.; Rohr, J. J. Nat. Prod. 2008, ASAP. [xxxvi] (a) M. Zhou and G. A. O’Doherty, Org. Lett. 2006, 8, 4339-4342. (b) R. S. Babu, Sanjeeva R. Guppi and G. A. O’Doherty, Org. Lett. 2006, 8, 1605 – 1608. (c) R. S. Babu and G. A. O’Doherty, J. Carb. Chem. 2005, 24, 169-177. (d) R. S. Babu, M. Zhou and G. A. O’Doherty, J. Am. Chem. Soc. 2004, 126, 3428 – 3429. [xxxvii] R. S. Babu, Sanjeeva R. Guppi and G. A. O’Doherty, Org. Lett. 2006, 8, 1605-1608. [xxxviii] (a) M. Zhou and G. A. O’Doherty, Org. Lett. 2006, 8, 4339-4342. (b) R. S. Babu, Sanjeeva R. Guppi and G. A. O’Doherty, Org. Lett. 2006, 8, 1605 – 1608.